Memristor technology, a groundbreaking concept first introduced by Leon Chua in 1971, has evolved into a vital component in the advancement of modern computing. These two-terminal devices are distinguished by their non-linear electrical characteristics and their ability to remember previous resistive states without power. This unique capability makes them particularly suited for neuromorphic computing, where they can simulate biological synapses, potentially revolutionizing memory and logic systems. With ongoing research into device fabrication, memristors are being tailored for energy-efficient computational tasks and complex scientific problem-solving, despite facing challenges in reliability and aging.
Key Takeaways
- Memristors are unique in their ability to retain resistive states without power, offering a blend of memory and computational functions.
- Their application in neuromorphic computing can mimic the synaptic functions of the human brain, leading to more efficient computing architectures.
- Advancements in memristor device fabrication, such as controlling IGZO thickness, are crucial for optimizing performance and energy efficiency.
- Memristor technology has the potential to disrupt traditional storage systems and could be instrumental in solving complex scientific problems with lower energy consumption.
- Despite their promise, memristors face challenges such as aging and reliability which must be addressed to ensure their future in mainstream computing.
Fundamentals of Memristor Technology
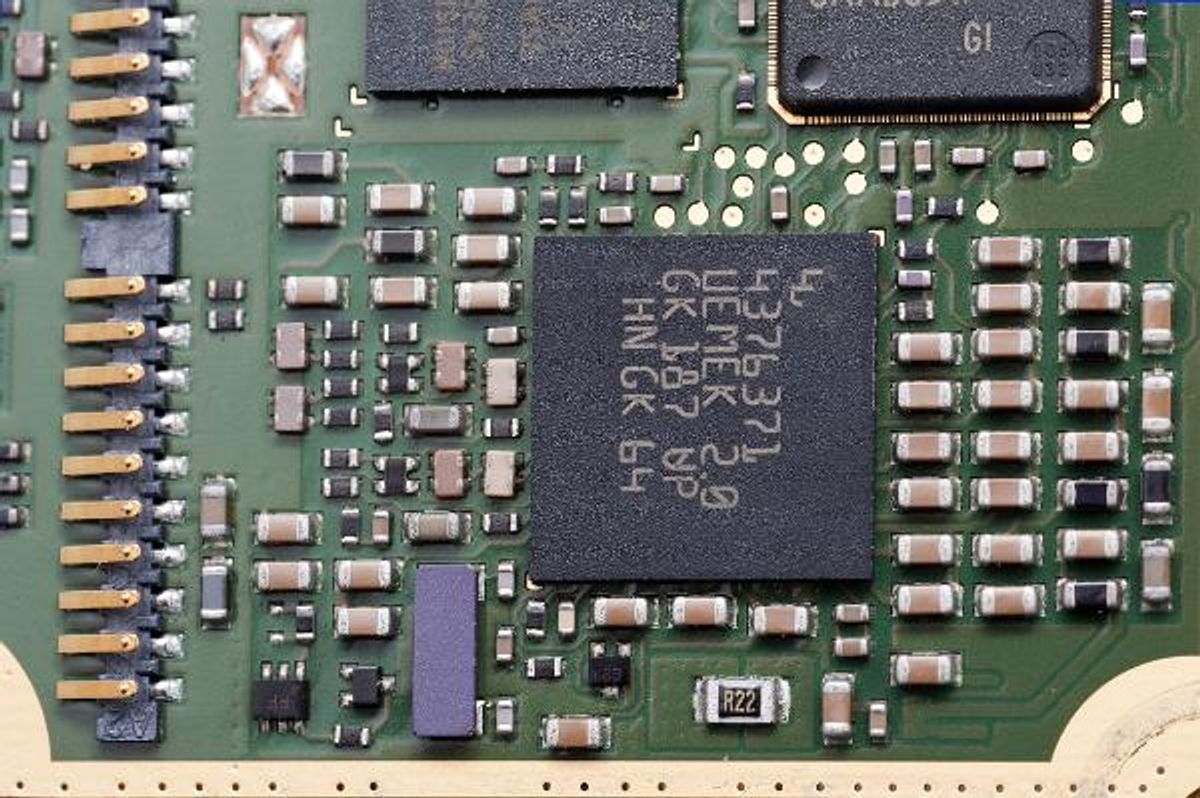
Defining Memristors and Their Characteristics
Memristors, conceptualized in 1971 by Leon Chua, are unique two-terminal electrical components that combine the properties of memory and resistance. In theory, memristors can be a nonlinear programmable analog resistor with memory properties that can take infinite resistive states. These devices are characterized by their ability to remember past voltages or currents that have passed through them, which is a fundamental aspect of their non-linear electrical characteristics.
Memristors can be broadly classified into two types: volatile and non-volatile. The non-volatile memristors maintain their resistive state even after the power is turned off, making them ideal for memory storage applications. On the other hand, volatile memristors require a continuous power supply to maintain their state, which can be advantageous for certain computational tasks.
Memristors show promise in expanding the development of neuromorphic computing, where they can simulate the synaptic behavior of the human brain.
The resistive states of memristors, such as the Low-Resistance State (LRS) and High-Resistance State (HRS), are influenced by the applied voltage and its duration. This characteristic enables memristors to be used as non-volatile memory elements, where LRS and HRS can represent binary logic states ‘0’ and ‘1’.
Volatile vs Non-Volatile Memristors
Memristors, as two-terminal devices, exhibit distinct behaviors based on their classification into volatile and non-volatile types. Volatile memristors are characterized by their inability to retain a resistive state without power; they revert to a high resistance state rapidly after the external excitation is removed. Non-volatile memristors, in contrast, maintain their resistive state even after the power is cut off, making them suitable for long-term data storage.
The ability of non-volatile memristors to preserve information lends itself to applications in artificial neural networks, where they can perform essential operations like vector-matrix multiplication. Volatile memristors, while not retaining information long-term, are still valuable in certain applications due to their quick response to changes in electrical bias.
The co-existence of threshold switching in volatile memristors and bipolar non-volatile switching in the same device allows for dynamic adaptation between modes, enhancing the versatility of memristor technology.
The following table summarizes the key differences between volatile and non-volatile memristors:
Property | Volatile Memristors | Non-Volatile Memristors |
---|---|---|
Data Retention | No (Reverts to HRS) | Yes (Maintains State) |
Response Time | Fast (Milliseconds to Nanoseconds) | Slower Compared to Volatile |
Applications | Quick Response Tasks | Long-Term Data Storage, Neural Networks |
The Role of Memristors in Memory and Logic
Memristors, with their ability to maintain a resistive state even when the power is off, are pivotal in the evolution of memory and logic circuits. Their unique property of remembering previous electrical states makes them ideal for non-volatile memory applications, where they can represent binary states ‘0’ and ‘1’ through High-Resistance State (HRS) and Low-Resistance State (LRS), respectively. This characteristic is not just beneficial for static storage but also for dynamic computing tasks, where memristors can perform logic operations within memory arrays, reducing the need for separate processing and memory units.
The integration of memristors in computational architectures allows for the development of in-memory computing, which is a paradigm shift from traditional computing models. In-memory computing leverages the memristive devices’ ability to execute matrix multiplication and addition operations, essential for processing complex data sets efficiently.
Memristive devices have gained significant attention as key components for in-memory computing due to their high-density arrays, rapid response times, and inherent computational capabilities.
Furthermore, the implementation of memristor-based logic operations, such as material implication, has been demonstrated, showcasing the potential for more compact and energy-efficient computing systems. These advancements suggest a future where memristor technology could play a central role in both memory storage and logic processing, paving the way for more sophisticated and integrated circuits.
Memristors in Neuromorphic Computing
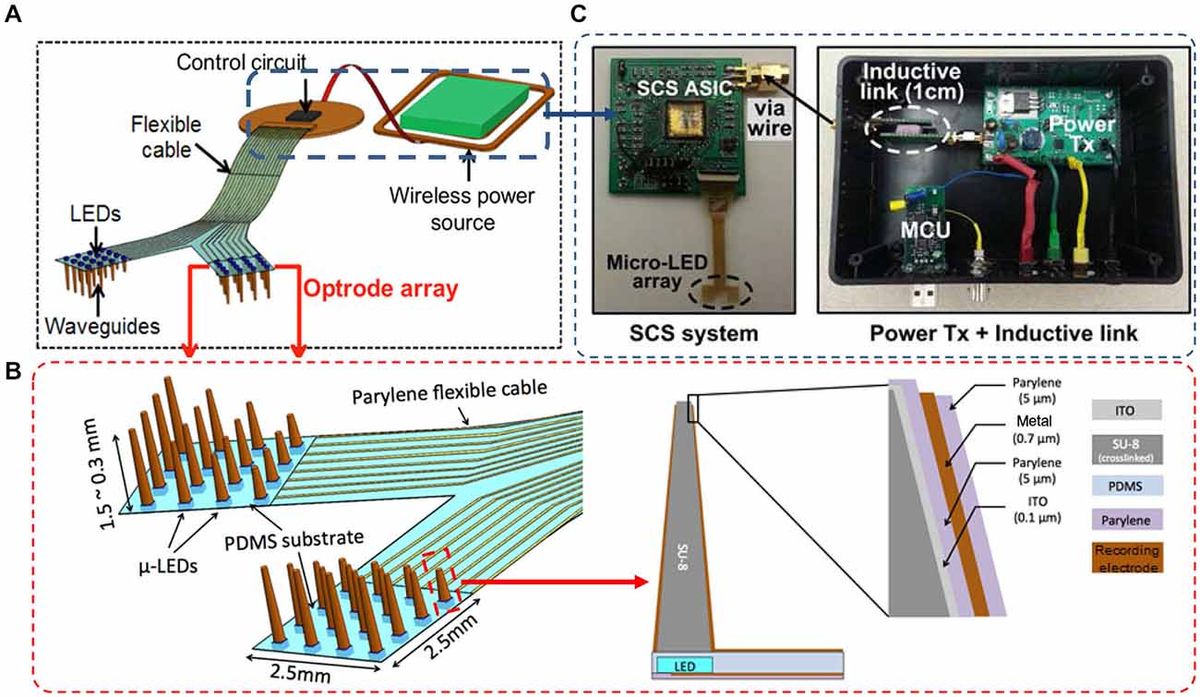
Simulating Biological Synapses
Memristors are at the forefront of simulating biological synapses, offering a promising avenue for neuromorphic computing. Their ability to emulate synaptic functions—such as long-term and short-term plasticity—is pivotal for creating more energy-efficient neural networks. By varying their resistance in response to electrical stimuli, memristors mimic the adaptive nature of biological synapses, which is essential for learning and memory in the brain.
Memristors enable the replication of complex electro-chemical dynamics of neurons, not only capturing the essence of neuronal efficacy but also the intricacies of neural communication.
The integration of memristors into neuromorphic devices allows for the construction of intricate networks that closely resemble the brain’s structure. These networks are composed of arrays of memristors, each representing a synaptic connection, and are capable of processing signals in a manner that significantly reduces power consumption compared to traditional computing systems.
- **Key Synaptic Functions Simulated by Memristors: **
- Long-term memory
- Weight multiplication
- Short-term memory
- Short-term plasticity
- Meta-plasticity
This approach not only conserves energy but also opens up new possibilities for artificial intelligence applications, such as image recognition and logic gate operations, by leveraging the inherent parallelism and adaptability of memristive networks.
Advancements in Neuromorphic Architectures
Neuromorphic computing architectures have made significant strides, leveraging the unique properties of memristors to more closely emulate the synaptic functions of the human brain. Memristors are pivotal in replicating the intricate network of synapses and neurons, providing a foundation for devices that operate with substantially lower power consumption compared to traditional digital AI computations.
The development of neuromorphic systems has been marked by several key advancements:
- Integration of memristor technology with existing CMOS circuits to create neurohybrid systems.
- Implementation of multichannel parallel processing, enabling more complex neural signal processing.
- Exploration of new materials, such as LiNbO3-based memristors, to enhance synaptic behavior and efficiency.
The compact structure of memristors allows for high-density circuits that can store and process information at the same physical locations, granting an opportunity for expanding the development of neuromorphic computing.
These advancements not only promise to reduce the energy and time required for data processing but also open new possibilities for applications ranging from biosensors to neuroprosthetics.
Potential and Limitations of Memristor-Based Neural Networks
Memristor technology has been pivotal in advancing neuromorphic computing, simulating the complex functions of biological synapses. Their ability to integrate storage and computation in high-density circuits mirrors the parallel computation capabilities of the human brain, offering a compact and efficient solution for artificial intelligence applications.
However, challenges persist in the deployment of memristor-based neural networks. Resistance drift and complex response behaviors necessitate circuit and algorithmic corrections, complicating design and impeding real-time learning. These limitations underscore the need for ongoing research to refine memristor properties for analog use in neuromorphic devices.
The promise of memristors in enhancing machine learning and neuromorphic hardware is tempered by on-chip training difficulties and the need for improved device integration.
Despite these hurdles, the potential of memristors to revolutionize computing remains significant. Continued exploration into memristor models and materials could unlock new possibilities for constructing artificial neural synapses and solving complex computational tasks.
Memristor Device Fabrication and Properties
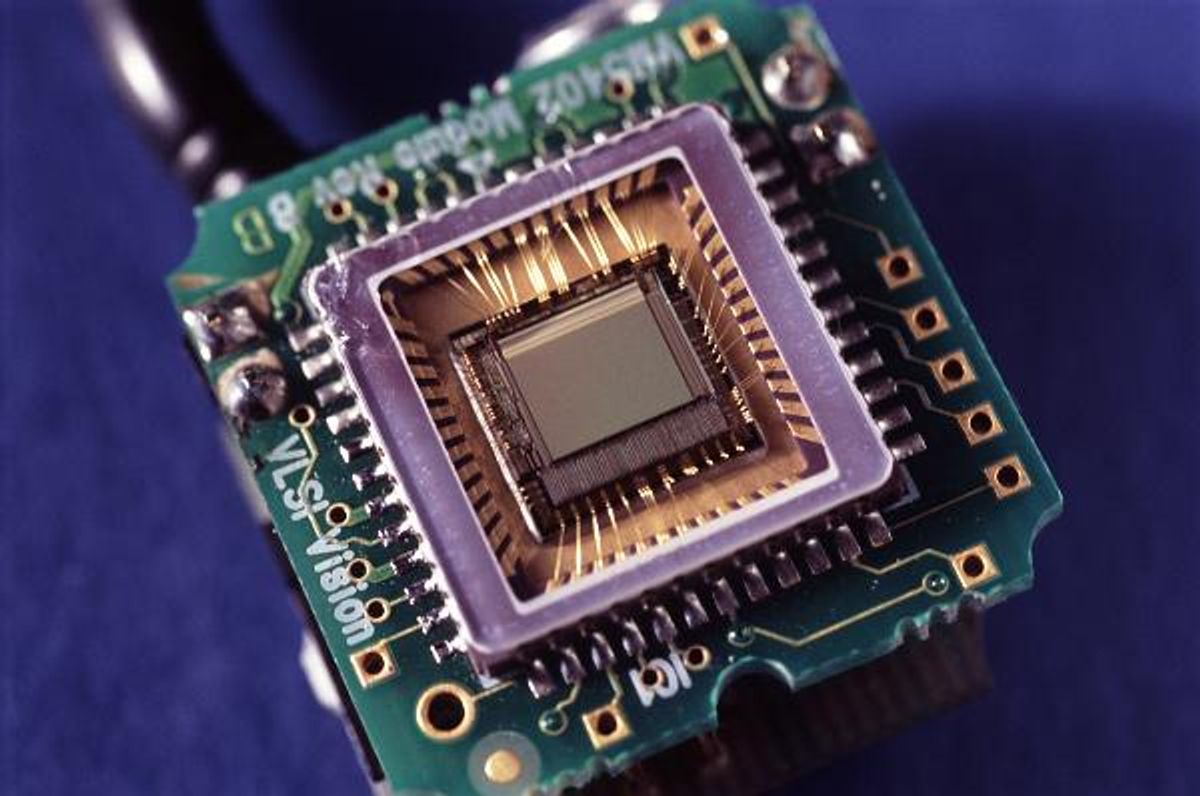
Influence of IGZO Thickness on Device Performance
The performance of memristor devices is significantly influenced by the thickness of the indium gallium zinc oxide (IGZO) layer. The optimized a-IGZO memristor demonstrates a notable change in resistive switching characteristics as the IGZO thickness varies. For instance, devices with thicker IGZO layers exhibit higher resistivity, which impacts the read current for both the low-resistance state (LRS) and high-resistance state (HRS).
The relationship between IGZO thickness and device performance is not linear, with both SET and RESET voltages increasing as the thickness grows. This indicates a complex interplay between material properties and electrical behavior.
The following table summarizes the observed changes in read current and voltage requirements based on IGZO thickness:
IGZO Thickness (µm) | LRS Read Current (mA) | HRS Read Current (µA) | SET Voltage (V) | RESET Voltage (V) |
---|---|---|---|---|
50 | 1.7 ± 0.2 | 300 ± 100 | 1 | -1.5 |
620 | 0.15 ± 0.09 | 0.7 ± 0.5 | 2 | -5 |
Additionally, a decrease in IGZO thickness leads to more gradual switching but also to a reduction in the memory window, which is a critical factor for the reliability of memristor-based memory devices.
Understanding Switching Mechanisms
Memristor technology has made significant strides in understanding the intricate processes that govern switching mechanisms. The switching mechanism in memristors is a critical factor that determines their performance and reliability. It involves the formation and dissolution of conductive filaments within the device, which can be influenced by various factors such as thermal resistance, ion mobility, and defect redistribution.
In the context of Valence Change Memory (VCM) cells, the nonlinearity and asymmetry of SET/RESET processes are closely associated with these factors. The devices exhibit a coexistence of volatile and non-volatile switching modes, allowing them to transition from a volatile to a non-volatile state by increasing the switching voltage, without the need for electroforming.
The coexistence of secondary switching modes within a single memristor cell often manifests as volatile behavior. This volatility can be attributed to oxygen exchange reactions at the interface with the active layer, such as a metal-oxide, and is influenced by the polarity of the applied voltage.
Understanding these mechanisms is essential for improving memristor performance and for the development of more reliable and efficient memristive devices.
Improving Energy Efficiency in Computational Tasks
As the demand for machine learning and artificial intelligence grows, the quest for enhanced computational efficiency is more critical than ever. Memristor technology is at the forefront of this revolution, offering a promising avenue for reducing power consumption and carbon emissions. This aligns with the broader goal of establishing a sustainable computational paradigm.
Recent studies have highlighted the potential of memristors in tackling the challenges of modern computing. For instance, memristors are being explored for their ability to solve complex equations that are currently straining digital computing systems. The inherent efficiency of memristors could lead to significant improvements in speed and energy consumption, which is vital for large-scale scientific applications.
The integration of memristors into computing architectures could transcend traditional limitations, providing a leap forward in computational capabilities. This is particularly relevant for computing-in-memory chips, which aim to support various computational tasks more efficiently on the chip itself.
Applications of Memristor Technology
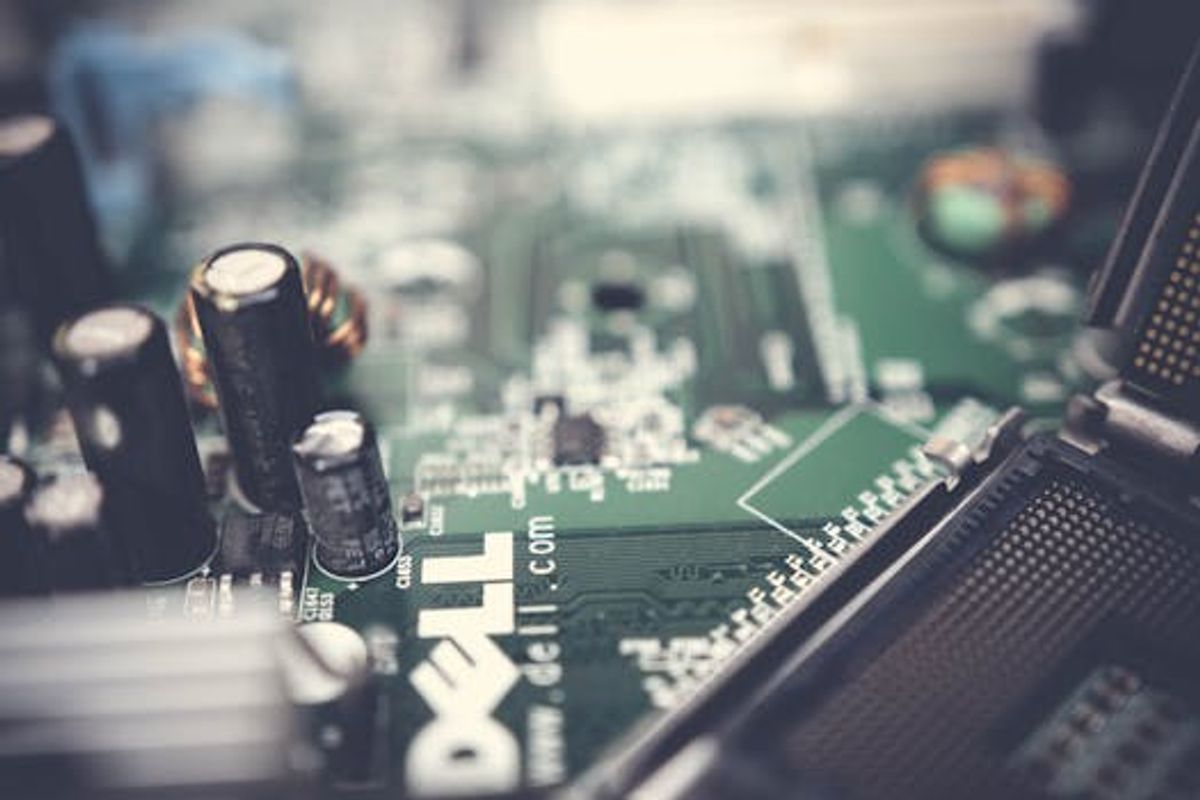
Storage Systems and Memory Hierarchies
The integration of memristor technology into storage systems has revolutionized the concept of memory hierarchies. Memristor-based storage systems offer a unique combination of speed, density, and non-volatility, making them ideal for a range of applications from embedded systems to large-scale data centers.
One of the key advantages of memristor storage is its ability to integrate with in-memory computing architectures. This integration facilitates near-storage data processing capabilities, enhancing design efficiency and reliability. For instance, a recent study highlighted a memristor-based storage system with convolutional autoencoder, which integrates data compression/decompression and multi-bit storage directly within the memory array.
The non-volatile nature of memristor crossbars allows systems to retain data even when powered off, providing significant energy savings compared to traditional SRAM or flash-based solutions.
The table below summarizes the benefits of memristor-based storage systems compared to conventional memory technologies:
Feature | Memristor-based Storage | Conventional Memory |
---|---|---|
Density | High | Moderate to Low |
Speed | Fast | Variable |
Non-volatility | Yes | No (SRAM) / Yes (Flash) |
Energy Efficiency | Improved | Less Efficient |
In-Memory Computing | Enabled | Limited |
As the technology matures, interdisciplinary collaboration will be essential to adapt these systems to diverse application scenarios and meet the requirements of various stakeholders.
Analog Computing and In-Memory Processing
Memristor technology is revolutionizing the way we approach computing, particularly in the realm of analog computing and in-memory processing. By leveraging the unique properties of memristors, systems can now perform computations directly within memory arrays, eliminating the need for data to shuttle back and forth between memory and processing units. This architectural shift promises to alleviate the bandwidth constraints that have long plagued traditional computing paradigms.
The integration of memristor technology into computing architectures enables a more efficient utilization of resources, mimicking the human brain’s ability to process information at the site of memory storage. This approach not only reduces latency but also significantly cuts down on energy consumption, a critical factor in the quest for sustainable computing solutions.
The benefits of in-memory computing with memristors are manifold, and they can be summarized as follows:
- Reduction in data transfer bottlenecks
- Enhanced computational speed and efficiency
- Lower energy consumption
- Potential for massively parallel processing
As researchers continue to explore and refine these technologies, the potential for memristor-based systems to transform the landscape of computing grows ever more promising. The challenge lies in fully harnessing these advantages while addressing the complexities of integrating memristor arrays into existing computational frameworks.
Solving Complex Scientific Problems with Memristors
The advent of memristor technology has opened new avenues for tackling complex scientific challenges. UMass Amherst research demonstrates the potential of memristors to revolutionize the field by performing computations with significantly less energy. This breakthrough addresses a critical limitation of traditional digital computing systems.
Memristors, with their ability to mimic the synaptic functions of the human brain, offer a unique approach to analog computing. Their nonlinear resistance, influenced by excitation history, enables them to integrate storage and computation, facilitating operations like matrix multiplication and addition. This dual capability is particularly advantageous for scientific applications that require intensive data processing.
Memristors grant the opportunity to build high-density circuits that are both energy-efficient and capable of complex computations. The inherent parallel computation capabilities of memristors make them suitable for advancing artificial intelligence and neuromorphic computing.
The table below summarizes the benefits of using memristors in scientific problem-solving:
Advantage | Description |
---|---|
Energy Efficiency | Reduced power consumption compared to digital systems |
Computational Power | Ability to perform complex calculations efficiently |
Density | High-density circuit integration for compact solutions |
Parallel Processing | Simulates human brain for advanced AI and neural networks |
Challenges and Future Directions
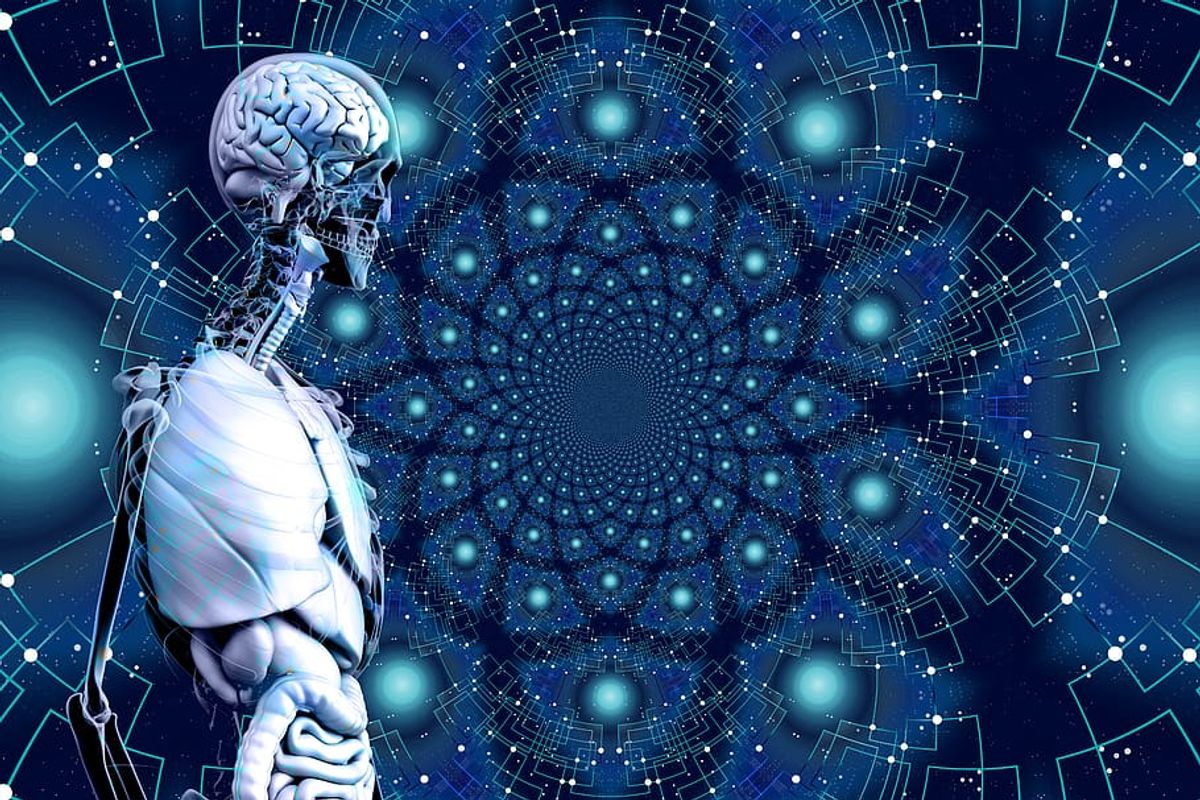
Overcoming the Hurdles of Digital Computing
As we delve into the era of big data and complex computational demands, digital computing systems are hitting a wall in terms of speed, energy efficiency, and infrastructure scalability. Memristor technology offers a promising alternative, capable of addressing these limitations by leveraging in-memory computing and analog processing capabilities.
Memristor-based systems can store intermediate data as conductance values within large crossbar arrays, streamlining operations like vector-matrix multiplication—a cornerstone of deep learning algorithms. This approach not only enhances computational efficiency but also circumvents the bandwidth constraints that traditional digital systems face.
By integrating memristor technology, we can significantly reduce the number of data transfers required, thereby minimizing energy consumption and improving overall system performance.
Furthermore, the intrinsic analog nature of memristor crossbar arrays eliminates the need for energy-intensive analog-to-digital and digital-to-analog conversions, offering a direct computational method that is both energy-efficient and effective for handling complex tasks.
Aging and Reliability Concerns in Memristor Devices
As memristor technology matures, aging and reliability emerge as critical concerns. Memristors are susceptible to resistance drift and stochastic behaviors, which can compromise device performance over time. These issues are particularly problematic for applications requiring high precision, such as neuromorphic computing.
The stochastic nature of memristors introduces variability in resistance and switching, known as Device-2-Device/Cycle-2-Cycle (D2D/C2C) variations. This variability, along with phenomena like Random Telegraph Noise (RTN), poses significant challenges for consistent device operation:
- Resistance variability
- Probabilistic switching
- Random Telegraph Noise (RTN)
- D2D/C2C variations
To mitigate these effects, circuit and algorithmic corrections are often necessary, leading to increased complexity in design and potential limitations in real-time learning capabilities.
Efforts to enhance the lifetime and reliability of memristor-based systems are ongoing. Strategies such as the ‘referencing-in-array’ scheme for RRAM-based computing architectures aim to address these concerns by providing more stable and predictable performance.
Innovations and Trends in Memristor Research
The landscape of memristor research is continuously evolving, with a significant focus on hardware implementation of memristor-based systems. These systems are not only pivotal for memory devices but also for the advancement of artificial neural networks, where memristors can mimic the synaptic behavior of the human brain.
Recent studies have highlighted the potential of memristors in beyond-complementary metal-oxide-semiconductor (CMOS) technologies. Their unique intrinsic properties make them a promising choice for future computing paradigms. The ongoing research is not without its challenges, as the ideal memristor is yet to be experimentally demonstrated, sparking both interest and controversy in the scientific community.
The integration of memristors into current technology stacks promises to revolutionize the way we approach storage and computing, offering a blend of high performance and energy efficiency.
Current trends in memristor research include the exploration of novel device mechanisms, the development of memristive crossbar arrays for storage and computing applications, and the enhancement of device longevity through aging-aware designs. These efforts are paving the way for memristors to solve complex scientific problems and to overcome the hurdles of digital computing.
Conclusion
Memristor technology, since its theoretical inception by Leon Chua in 1971, has evolved into a promising component for the future of computing. With their unique ability to mimic the synaptic functions of the human brain, memristors offer a new paradigm in neuromorphic computing, potentially revolutionizing the way we approach data storage and processing. The non-linear resistance and memory functions of memristors enable them to perform complex operations with lower energy consumption, addressing some of the major limitations of traditional digital computing. Despite the challenges that remain in the fabrication and application of memristor devices, the advancements in this field suggest a bright future where memristor-based systems could lead to more efficient and intelligent computing solutions. As research continues to unravel the full potential of memristors, it is clear that they hold a significant place in the evolution of electronic components and the development of next-generation computing technologies.
Frequently Asked Questions
What is a memristor and how does it function?
A memristor is a non-linear two-terminal electrical component that relates electric charge and magnetic flux linkage. It functions by changing its resistance based on the history of voltage applied and current passed through it, effectively ‘remembering’ its past states.
What are the differences between volatile and non-volatile memristors?
Volatile memristors lose their resistive state when the power is turned off, similar to RAM in computers. Non-volatile memristors maintain their resistive state even without power, akin to flash memory, making them suitable for long-term data storage.
How do memristors contribute to neuromorphic computing?
Memristors can simulate the synaptic functions of biological brains, allowing for the creation of neuromorphic computing systems that mimic neural networks. They can integrate storage and computation, performing operations like matrix multiplication and addition.
What impact does IGZO thickness have on memristor device performance?
The thickness of IGZO (Indium Gallium Zinc Oxide) in memristor devices influences their memory window and switching voltage, affecting both their storage capacity and the energy efficiency of their switching operations.
What are some applications of memristor technology?
Memristor technology can be applied in various fields such as non-volatile memory systems, analog computing, in-memory processing, and solving complex scientific problems by providing energy-efficient computation and storage solutions.
What are the current challenges and future directions in memristor research?
Challenges in memristor research include overcoming the limitations of digital computing, addressing aging and reliability concerns, and continuously innovating to improve device performance. Future research is focused on developing novel architectures and applications.
Discover more from TechResider Submit AI Tool
Subscribe to get the latest posts sent to your email.